The propeller-driven six-seater churns straight toward the brown plume over Eyjafjallajökull, unlike other aircraft taking off from Reykjavík airport. Inside, accompanied by a seasoned pilot, sits Björn Oddsson, a graduate student at the University of Iceland, entrusted with an infrared sensor derived from military bombing systems. But the only bombs Oddsson talks about are the lava boulders erupting from the volcano 80 miles away.
As they approach the volcano Oddsson opens the window so that the infrared sensor can function properly. A frigid wind whips in, chilling the cabin to near-Arctic temperatures, but Oddsson doesn’t mind; he is focused on calibrating the temperature scale on the device. The sensor, which looks like a video camera, is still relatively new and he’s eager to get it right. His supervisors expect him to report his findings at a briefing the next afternoon, April 19, the sixth day of the present eruption.
At the time of the flight, ash from the Eyjafjallajökull eruption had grounded north Atlantic air traffic for four days; by one estimate, $1.7bn was lost by commercial airlines by the end of the first week. Across Europe, the disruption led to a reassessment of aviation rules and the reliability of wind forecasts. Scientists were called on to advise – and called to task; was it really impossible to fly through this stuff? How reliable were the predictions about the ash’s drift? What was the evidence?
Those questions have few quick answers, and at the root of the problem are other questions: why did this eruption produce ash while an earlier one from the same volcano didn’t, how long might it keep producing ash, would there be warnings of future eruptions? The chemistry of the magma, the shape of the volcano, how much ice covers it – all these factors determine whether or not a volcano produces ash, the explosiveness of its eruptions and how high the ash will fly.
Weeks before the volcano seized the attention of politicians, on March 20, when the current series of eruptions began, volcanologists flocked to Eyjafjallajökull to sample rocks, gas and glacier runoff and make flyovers with radar and infrared instruments. I tagged along on two field trips, sat in on discussions with dozens of Icelandic researchers, and interviewed others from Switzerland and Hawaii. In doing so, I got a peek over the shoulders of scientists into the cloudy crystal ball that predicts our flying future.
. . .
The conference room on the third floor of the University of Iceland’s natural sciences building was a cauldron of excitement ahead of Oddsson’s briefing. A large map of southern Iceland hung on one wall, and coffee-slurping, laptop-toting researchers gathered in front of a projection screen, sitting on the floor when all the seats were full.
Magnús Tumi Guðmundsson, Oddsson’s supervisor and one of the university’s senior researchers, started the meeting without ado, introducing Oddsson, who switched on a video of his flight. “There’s not as much interaction between water and magma as before,” Oddsson told us. Colourful blobs erupted silently on the screen behind him against a black background. It looked a little like an Atari video game circa 1983.
Water and magma can be a powerful engine for ash clouds, because when they meet, the ice-cold water shatters the red-hot magma. Percussive detonations of awesome violence send the minuscule shards skyward. Ash from the April eruption through Eyjafjallajökull’s icecap hitched a ride higher into the atmosphere than that from the earlier eruption, on its rocky shoulder, thanks in part to the billowing steam. It was like a sauna writ large.
All this occurred during the first few days of the present eruption, melting the icepack on top of the vents and flooding roads below the volcano. By the end of the first weekend, the meltwater had slowed to a relative trickle. “I was trying to explain to my mother how it’s working now,” said Oddsson. “And she said it sounded like a broken toilet. It’s not flushing any more, it just has a constant leak.”
Oddsson is not the only scientist making forays to the volcano. Every other day, the Icelandic Coast Guard sends a radar-equipped aircraft into the river valley alongside the volcano, mapping the shifting land even through clouds. Other researchers make regular forays into the shadow of the volcano to collect lava, ash and river-water samples, using chemical sniffers to monitor gasses seeping out of the ground. Geophysicists, meanwhile, monitor data from the seismic and global positioning system (GPS) stations they had positioned on the mountain’s slopes months before. Centimetre-long shifts had warned them that magma was swelling beneath the skin of the volcano – never a good sign.
Ash clouds ahead?
Not all volcanic explosions throw up flight-impeding ash. Here’s a step-by-step guide to the questions that volcanologists ask – and an explanation of how their answers might affect your travel plans
1. Where’s the magma coming from?
Magma that has been lingering near the surface tends to grow more viscous – and create more explosive eruptions – than magma shooting up straight from the earth’s mantle. Viscous, explosive magma is bad news for fliers: it tends to lead to billowing ash.
Scientists follow this using seismic stations and GPS sensors: they tally up the volume of magma close to the surface of a volcano by listening for telltale earthquakes as the magma enters from the mantle and then detecting when the magma makes the volcano’s skin stretch.
2. What kind of gases preceded it?
Answering this can help scientists with the previous question. Sulphur dioxide and carbon dioxide, among other gases, are released from magma at different pressures. And pressure translates into depth, so scientists use the gases as a precursor sign of where the magma is and what it’s made of (see question 4).
Scientists can use chemical sniffers such as gas chromatography to detect particles in the air coming out of a volcano, and spectrometers which read a gas’s optical signature against a bright light source, such as the sun.
3. What sort of surface is it breaking through?
This one’s pretty simple. If an eruption shatters an ice cap, you get ash. If it doesn’t, it’s much less likely: witness the earlier Eyjafjallajökull eruption. Grímsvötn regularly erupts without incident underneath the largest icecap in Iceland. Whether an ash cloud will get in the way of air traffic is another question – one for the meteorologists.
4. What’s this magma made of?
If you take a look at the chemical make-up of the volcano’s tephra – the debris from airborne magma – you can get a sense of how viscous or explosive any magma yet to come might be.
This involves a trek to the volcano by researchers, who collect tephra by the bucketload and bring it back to the lab. The chemicals they’re looking out for are silica, a signal that the magma will be stickier and more explosive, and magnesium, which hints at more fluid magma that dribbles out of the mouth of the volcano.
5. How long has the eruption been going?
The magma from close to the earth’s surface tends to get spewed upward first, so the beginning of an eruption is more likely to release ash than its later stages.
If it’s a well-monitored, straightforward volcano (in Iceland, Katla is, Eyjafjallajökull isn’t), scientists will know roughly how much viscous magma it’s storing near the surface; from there, just have a glance at your calendar.
Sigrún Hreinsdóttir is one of the University of Iceland geophysicists using GPS stations to monitor swelling. Employing simple physics and highly sensitive GPS systems, she tracks tiny movements of the volcano’s surface. Then she tries to estimate how much magma is accumulating beneath the volcano, as if calculating a person’s lung capacity by how much their chest rises when they breathe.
One of Hreinsdóttir’s GPS stations, located on a farm on the volcano’s flank, stopped transmitting data over the weekend and she frets that the eruption disabled it. “Maybe the farmer just turned off his power,” she says hopefully. Luckily, she has enough data from the past few months to show that Eyjafjallajökull has accumulated between 40 and 60 million cubic metres of magma starting in January. Lots of magna – but small potatoes among volcanoes. Knowing how much magma a volcano stores in its chamber is a bit like knowing how many bullets there are in an enemy’s gun. Eyjafjallajökull, it appears, is packing relatively little heat.
But volcanoes can reload. The newly detected material isn’t emerging from the volcano yet: it’s just pushing up older material that had rested in the chamber before. “We don’t know when [the currently erupting] material came in,” Hreinsdóttir explains.
Why would air travellers care whether it’s “new” magma or old spurting from the volcano? Magma changes as it nears the surface because there is less pressure and less heat than in the mantle – the layer of the planet between surface and core – where it originates. It grows more viscous, and more explosive, than magma that shoots up straight from the mantle, like the photogenic material from the March eruption – which came from a different vent. So when the volcano runs out of the stale magma closer to the surface, its eruption could grow less explosive and send up less ash.
Back at the briefing, the scientists politely asked the three journalists present not to repeat what they say as they hash out what their daily bulletin should include. But it’s fair to report that when this many different streams of data get into the hands of this many scientists, disagreement ensues. Some researchers are willing to say that the eruption, which is already weaker than on the day it first shut down air traffic, is unlikely to grow larger. Others point out that they still don’t have a handle on how much or how fast magma from the mantle might continue to feed the intermediate chambers beneath the volcano. “Eyjafjallajökull doesn’t seem to be typical,” Hreinsdóttir notes later.
One contrarian researcher pipes up to say that if the magma grows more viscous, “something horrible” could be on the way. Nobody says they agree with him, but nobody contradicts him, either.
. . .
Geophysicist Michael Poland is familiar with the uncertainty of volcano briefings. He works at the US Geological Survey’s Hawaiian Volcano Observatory (HVO), on Kilauea, which has been erupting with mostly runny magma since 1983, with intermittent bouts of more explosive activity. Poland attends weekly briefings with colleagues at HVO, where, he says, “we try to put together the story” from many strands of data.
As well as tracking GPS sensors, Poland studies radar images of Kilauea taken by satellites. By comparing images, he can detect deformation – swelling – over a larger area, though with less precision, than the GPS stations cover. But ever since a geochemist colleague successfully predicted an eruption of Kilauea in 2008 based on sulphur dioxide emissions, Poland has taken an interest in gases that emerge just ahead of the magma and reveal clues about it, such as its depth and how fast it is rising. Such clues are complementary, Poland says: “The best predictive value comes from all these systems together … not so dissimilar to weather forecasting.”
Another research group compared gas emissions to deformation during an eruption on Stromboli in Italy and published a study in 2007 revealing the depth at which explosion-inducing gas bubbles were separating from the main magma flow. That study’s lead author, Mike Burton of the Istituto Nazionale di Geofisica e Vulcanologia in Catania, says that team’s goal is to work out “reasonable relationships” between gas emissions, deformation and eruptive activity.
On another sunny volcano on the island of Nisyros, near Rhodes, Greece, Volker Dietrich of the Swiss Federal Institute of Technology in Zurich is also trying to integrate what he matter-of-factly calls “a hell of a lot of data”. Dietrich was one of the principal researchers on a three-year European Union-funded project to create a computer program that could combine a dozen different sources of monitoring data, ranging from oceanographic surveys to satellite imagery to CO2 emissions, into a single graphic model of the volcano’s activity.
There are ad hoc systems like the one in Hawaii at volcano observatories in Italy, Japan and elsewhere, Dietrich explains, “but an integrated [analysis] system – nobody has it.” Yet his project ended in 2003, and when he proposed a more ambitious computer program that would activate an array of monitors when early-warning sensors detected volcanism, the proposal went unfunded. Instead, scientists such as Guðmundsson, who are expected to interpret a volcano’s behaviour for safety officials and politicians, must listen to a roomful of experts, each bringing a different type of data to the table, and try to identify a tune in all the noise.
. . .
Despite their webcams and satellites and seismic stations, volcano researchers still prefer one method of data collection to all others: going out in the field and cracking open freshly made rocks. They call it “ground truth” in an oblique sneer at remote sensing methods.
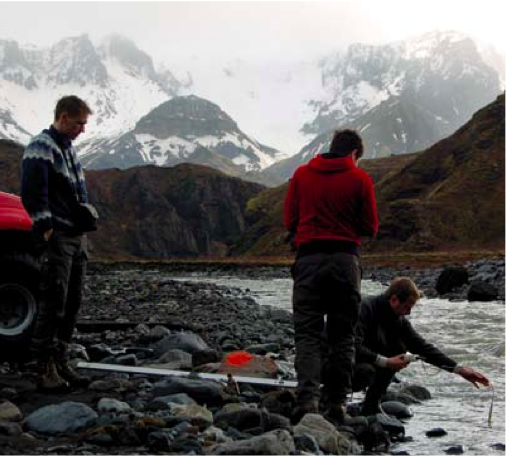
What are they looking for? The chemistry of the tephra – freshly erupted debris – can tell us a little about the likely consistency of the next batch of magma. The more silica in the tephra, the more sticky – and therefore more explosive – the magma will probably be. More magnesium in the tephra indicates more fluid magma.
One sunny morning between Eyjafjallajökull’s March and April eruptions, I accompany a truckload of scientists as they ford the many braids of the Markarfljót, a glacial river running along the northern foot of the volcano. The researchers get out of the truck at the steaming mouth of a mossy canyon whose upper half contains lava still hot from the March eruption.
Halfway up the canyon, a German television reporter falls into the sulphurous river, last measured at around 50°C, just a few degrees warmer than Reykjavík’s outdoor hot tubs. She is lucky. The water is 81°C by the time the team reaches the lava front. There, it looks as if a fleet of dump trucks has deposited tons of scorched rubble on top of the river, which trickles half-heartedly underneath and alongside the steaming black lava.
A rock hammer rings out in the steam-muffled air as two geologists break open a lava rock. They curse as it burns their hands through wool mittens and ooh and aah when they find tiny green crystals inside. But they don’t shout Eureka, nor do they appear to know exactly what the crystals say about the eruption. Back in their labs, the scientists will patiently reverse-engineer the chemistry of the magma which formed the lava rock and calculate the speed with which it emerged. This will strike one small note in the cacophony of data. This, I reflect on the walk back out, is the way science works – in broken tones and scattered phrases, rarely in time with the batons of governments’ would-be conductors.
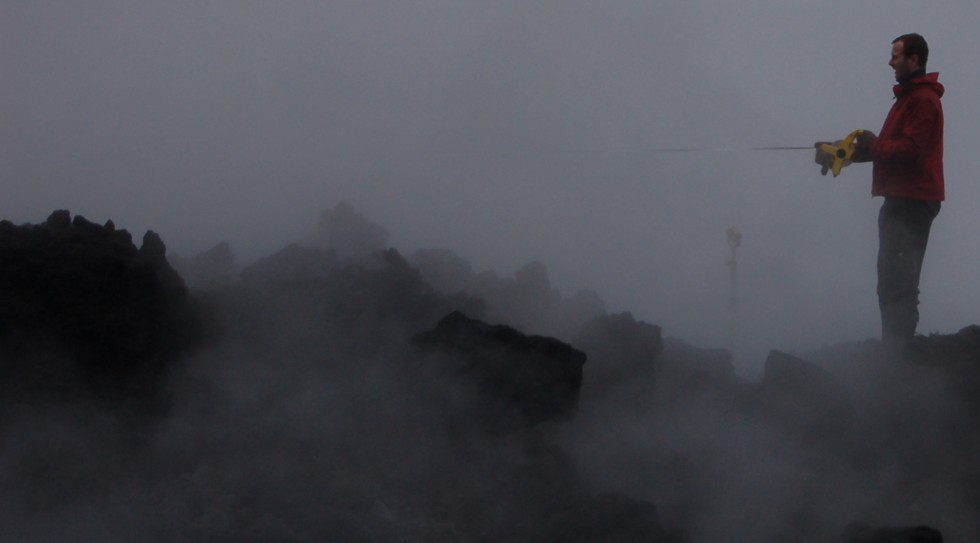
. . .
Amid all the chaos of Eyjafjallajökull’s eruption, some people have warned of worse to come, in the form of other, bigger volcanoes. After all, an Icelandic volcano called Laki erupted in mid-1783, filling Europe’s skies with sulphur dioxide and fluorine-laced ash that hurt agricultural yields for two years (but didn’t stop the flight of the first hot air balloon over Paris later that year). Even Iceland’s president, Ólafur Ragnar Grímsson, got in on the act, warning the BBC in April that Eyjafjallajökull was just a rehearsal for its bigger neighbour, Katla. His words, which some Icelanders blame for a drop-off in the volcano-tourism industry, were also unwelcome among Icelandic scientists.
“Katla can erupt but it really doesn’t have to be much worse than we’ve seen,” Oddsson explains. “We’ve seen a lot.” He cites his supervisor, who has been telling any foreign journalist willing to listen the good news that Katla typically erupts in a smaller way after big eruptions and that its last eruption, in 1918, was a whopper. Of course, volcanoes are not bound by patterns, but, for all Iceland’s researchers know, the next eruption of Katla won’t even break the icecap – not all do. No broken icecap means no ash cloud. Still, “it will erupt,” Hreinsdóttir acknowledges, and when it does, the better-studied volcano’s many seismic stations and GPS sensors could give several days’ warning. Scientists may be able to advise people living nearby to evacuate and aviation authorities to keep an eye on the wind forecast.
Unlike Eyjafjallajökull, Katla’s magma chamber is relatively well understood. “Eyjafjallajökull is not a simple volcano, which is what makes it so interesting,” Hreinsdóttir says. All the scientific and public attention it has attracted should be helpful in the long term for understanding other volcanoes. Of course, Dietrich adds, in some cases the clues will simply come too late: unpredictability, at least some of the time, is also natural.
That won’t stop politicians and journalists from inventing endings to Eyjafjallajökull’s story. When Icelandic journalists visited the volcano in mid-April they compared the darkness of the ash cloud to Ragnarök, the final pitched battle of the Norse gods during which cosmic wolves will swallow the sun and moon. But unlike in the apocalyptic Norse myth, Iceland and the rest of Earth’s bubbling, steaming surface will continue to erupt, in both minor and major keys. And today’s scientific soothsayers are listening closely to the volcano instead of foretelling endings they cannot guarantee.
Today, for example, the daily bulletin from the University of Iceland’s Institute of Earth Sciences finishes on the same note it has every day since late April: “There are no signs that the eruption is about to end.”
This feature first appeared in the Financial Times Weekend Magazine [html] [pdf].
The FT’s Dear Economist column cited the story on 12 June 2010: [html].